Translated into English by Patricia Lima Carlos.
When we study dynamics (physics) we learn that there are three ways for a body to be in static equilibrium and that are shown in Figure 1.
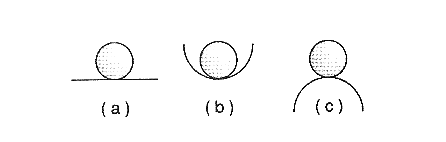
In the first condition we have the so-called indifferent equilibrium (a), because in any position of the plane in which the sphere is placed, it can certainly be stationary, without problems, in a condition of static equilibrium. In the second, we have a stable equilibrium condition (b) which is achieved only in the lowest position of the gutter. If we try to remove the sphere from this position by placing it in another, it does not stay and tends to return to its original position. Finally, we have an unstable equilibrium condition (c) which is precisely what will serve as the starting point for the study of our electronic problem.
In this condition the sphere is balanced, but very critically in the indicated position. However, any movement, however slight, to one side or another that tends to displace the sphere of this position, causes forces to take place that take that sphere away from the equilibrium to never come back spontaneously . In electronics there is a phenomenon that can be analyzed in a similar way: the thermal drift.
TAKING IT TO ELECTRONICS
All electronic components are very sensitive to temperature changes. No matter how small, changes in temperature tend to affect markedly the characteristics of most components. Transistors, diodes and semiconductors in general, have their leakage currents increased significantly when the temperature of their junctions increases, as shown in Figure 2.
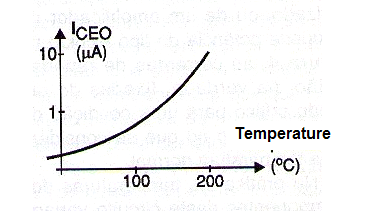
In other words, the inverse resistance of the semiconductor junctions decreases as the temperature increases. However, components, such as a wound wire resistor, have positive temperature coefficients, that is, their resistance increases as the temperature increases. But, we can also write about the NTCs (Negative Temperature Coefficient) which are components whose resistance decreases with increasing temperature, as shown in Figure 3.
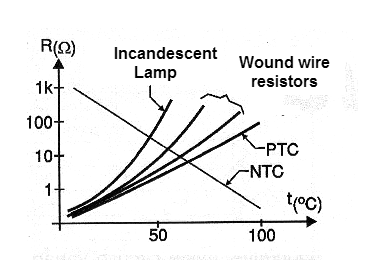
In an electronic circuit such as an audio output stage of a transistorized little radio or a low power amplifier of the type shown in Figure 4, the quiescent currents are in fact critically fixed to an operating condition in the what is considered a normal temperature.
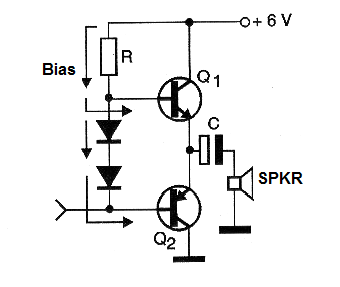
In practice the component temperatures of this circuit vary, either in function of the temperature of the places where they work or by the heat generated by itself which depends on the way of its operation. When required at full power, the transistor tends to generate more heat and thus heat it to the point of changing the optimal operating conditions of the circuit itself.
Like the sphere in the equilibrium conditions we take as an example, the operation of such a stage can tend to three conditions. The components may have such characteristics and be connected in such a way that, regardless of the operating temperature (within a range of values ??that does not imply in their destruction), any increase in a resistance is compensated by altering another in order to keep constant currents and therefore the polarization of the circuit.
In this case, the amounts of heat generated by the components are not altered and the thermal equilibrium of the device can be considered as indifferent. The complexity of most circuits, both in terms of temperature rise and number of components, and the variety of behaviors that are not linear with the temperature makes this condition very difficult to be obtained.
See that it would be interesting to have a device whose operating characteristics were totally independent from the ambient temperature, because the problems that we are analyzing in this article would not occur. However, what becomes dangerous to the integrity of a device is that we can have an unstable equilibrium condition. Take, as an example, an output stage of a push-pull audio amplifier, as shown in Figure 5.
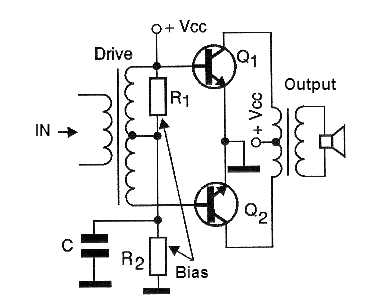
The components which polarize the bases of the transistors are calculated to a value that produces a resting current that does not compromise the output transistors and which at the same time, with the implementation of an audio signal, we have an amplification with the desired performance and fidelity .
Let us suppose, however, that for some reason the amplifier is driven into operation at a temperature higher than expected. This can be further aggravated by a poor ventilation condition (someone placed a few discs just covering the ventilation holes of the device on the case, which is very normal for this type of device).
As the temperature rises, the leakage current of the transistors increases with the base current. The result is that the collector current is determined by the base current and with the increase of the first current, the result is an increase of the collector current in the standby condition. The increase in the collector current has an important consequence: it makes the transistor generate more heat, and it has to dissipate this heat.
Now, to dissipate more heat, the transistor heats up more and the result of the additional temperature rise could not be otherwise: it increases the leakage current that is added to the base current. The effect is similar to that of a "snowball", increasing the base current increases the collector’s, increasing the temperature and again the base current and the final result could not be otherwise: the current in the component becomes so intense, the heat generates the burning which is inevitable! See, then, that an initial "push" is enough for the process to take shape, with a "thermal drift" which makes the circuit escape the ideal operating conditions causes the most sensitive components to burn. For a circuit like this one it is necessary to add resources that prevent this phenomenon from occurring.
A simple way of compensating for the effects of temperature rise which tends to increase the current in the transistors is achieved with the use of a thermistor or NTC, connected as shown in Figure 6.
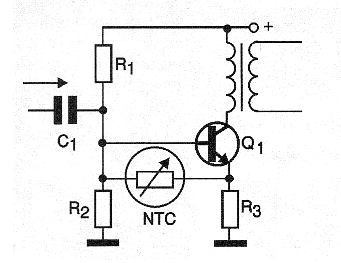
The thermistor or the resistor with NTC (Negative Temperature Coefficient) is a component that, as the name says, decreases resistance when the temperature increases. Connected between the base of the transistor and the emitter (through the transformer winding) it tends to decrease the polarized voltage and thereby reduce the base current when the temperature rises.
However, this causes the total current in the transistor to retain itself and it does not tend to overheat. In high power amplifiers with connected transistors in the configuration in complementary or quasi-complementary symmetry, we have two other possibilities to keep the thermal equilibrium and thus avoid technical drift. These possibilities are shown in the circuitry of Figure 7.
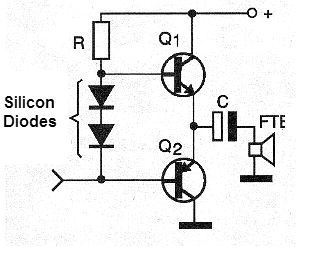
Common silicon diodes are used to bias the bases of the transistors such that their resistance decreases with the temperature rise. In fact, what happens is a rise in current in these components when the temperature increases and thus we have a similar effect to those obtained by NTCs. The great advantage of this configuration is that the diodes intrinsically tend to remain between their terminals the same base voltage - emitter that we need to bias the transistor.
Some high power amplifiers do the same, but using a low power general purpose transistor as a sensor, as shown in Figure 8.
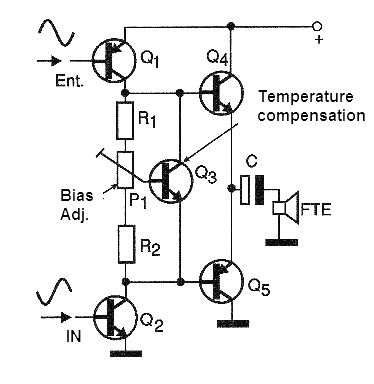
In order to "feel" the temperature of the output transistors, which are precisely the ones that must dissipate more heat and therefore operate at the limits leading to thermal drift to be an element of great danger to their integrity, the sensor assembly (transistor) is made in the heatsink itself, as shown in Figure 9.
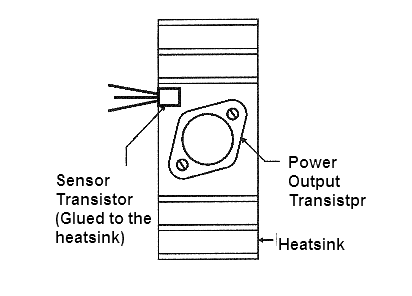
The transistors used as sensors in this case are glued with epoxy or another strong glue on the heatsink of the output transistors of the amplifier. The operation of this "safety system" is simple: when the temperature increases and the collector current of the power transistors also tends to increase; also increases the conduction of the transistor used as a sensor, thereby diverting the base current which is automatically reduced.
In these conditions, the polarized current decreases, also reduces the current between the collector and the emitter and with it the power developed in the transistor, responsible for the raise of its temperature. But, it is not only with transistorized circuits that this problem can occur. In fact, with integrated circuits, the problem can be considered even more serious because all the components are in the same silicon chip and any excessive heating of one of them is immediately reflected in the characteristics of the others.
This way, special care is taken in the designs, especially of the integrated circuits that work with high currents, in the way of adding internal thermal protections which avoid the problem of the drift, compensating any problem of excessive increase of currents by the raise of the temperature. Integrated circuits voltage regulators, for example, have internal configurations which prevent the current from rising if the temperature rises and in some cases they even cut off the output current when this temperature exceeds a value considered dangerous. 7805, shown in Figure 10 is an example of an integrated circuit with these features.
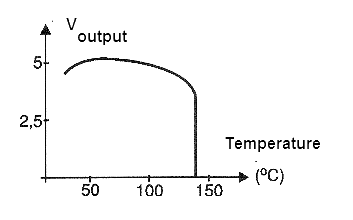
For integrated audio amplifiers, we also have internal protection circuits against the problems caused by thermal drift. These circuits avoid burning in case of a tendency to increase currents that would cause excessive heating of the component. There are additional features for the microprocessors, which normally work in the limit conditions of heat dissipation.
Sensor systems detect abnormal temperature rises, shutting down the sectors that are responsible for generating heat or reducing the speed of operation in a way that less heat is generated.
CONCLUSION
By obtaining a condition seemingly ideal of operation from a circuit at ambient temperature, we can not always guarantee that the project is ready for use in a range of temperatures we normally encounter on a daily basis. High ambient temperature or even over-normal circuit temperature caused by ventilation problems or prolonged operations can be the source of big problems for a circuit.
A simple "push" in order to remove from the circuits the conditions of rest, which keeps its stability of operation, can cause the system to leave the control with the current increase in dangerous points and this can culminate with the burning of components. Devices which have stages that operate at high power are the most critical, but that does not mean that others are immune. If the polarity equilibrium of a circuit is critical, precautions should be taken to obtain proper compensation. Without this, thermal drift can lead to losing the best of the projects.